LIGOS’ detection is the most precise measurement ever made in human history and marks five milestones for physics.
On February 11, 2016, the National Science Foundation (NSF) announced the detection of gravitational waves by the Laser Interferometer Gravitational-Wave Observatory (LIGO), a pair of ground-based observatories, one in Livingston, Louisiana, and the other in Hanford, Washington.
It was a landmark historic event which is leading astronomers to call this “the century of gravitational wave astronomy.”
LIGOS’ detection is the most precise measurement ever made in human history and marks five milestones for physics as the first direct detection of:
- gravitational waves,
- a black hole,
- a binary system of black holes,
- the merger of black holes, and
- a spinning black hole.
A century ago, Albert Einstein postulated the existence of gravitational waves.
In 1916, a century ago, Albert Einstein postulated the existence of gravitational waves as part of his theory of general relativity. Direct evidence of these waves was the last remaining prediction to be observed of Einstein’s theory of general relativity.
Einstein predicted that masses deform space-time, that hard-to-visualize environment that combines time with our familiar three-dimensional space (length, width, and depth). The change of position of a massive object will cause a distortion in the four-dimensional mesh of space-time. As a massive object moves, it takes space-time some time to react to that motion. This distortion or ripple due to the reaction of space-time will propagate at the speed of light, resulting in gravitational waves, similar to seismic waves on the earth crust produced by an earthquake.
Due to the enormous distances between us and the likely sources of gravitational waves, Einstein expected gravitational waves to be too faint to ever be detected. Indeed, the detection of gravitational waves in itself is a technological breakthrough, achieved with one of the most complex detection systems ever built.
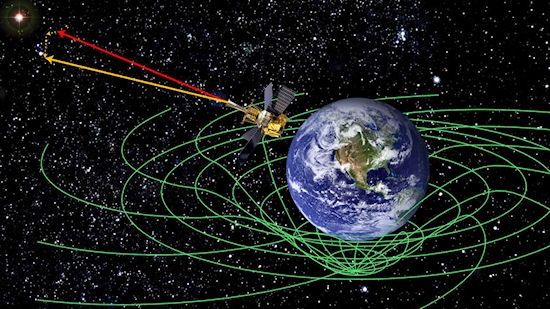
Artist’s conception of the curved space-time around Earth. Image from NASA Science News.
Each of LIGO’s twin observatories is an L-shape tunnel that houses an ultrasensitive detector developed by the California Institute of Technology (Caltech) and the Massachusetts Institute of Technology (MIT). Each arm of the L-shaped tunnel measures 2.5 miles and the observatories are 1,875 miles apart. Each arm consists of layers of steel and concrete, and an interior of nothingness: 2.5 million gallons of empty space. At the end of each arm there is a mirror hanging by glass threads, isolated completely from the environment.
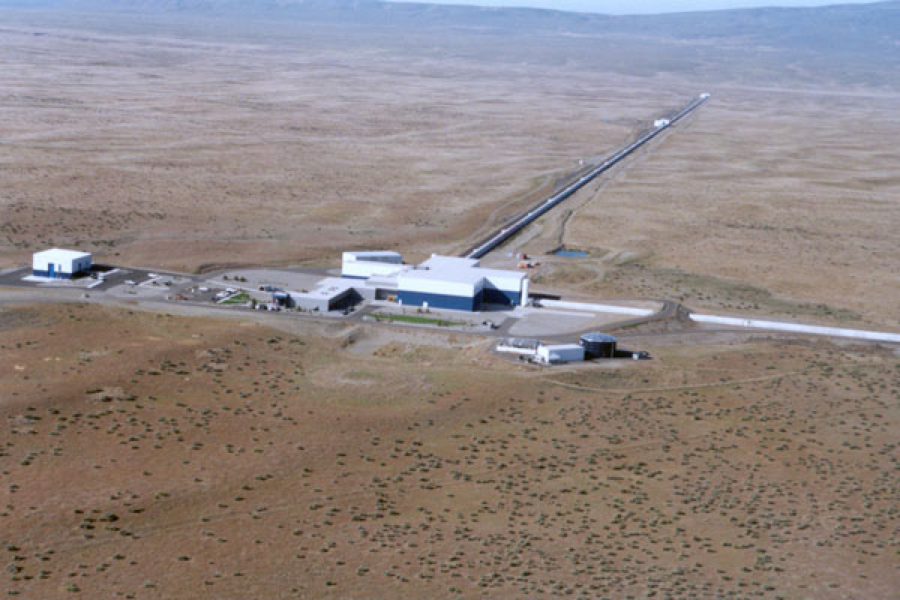
Observatory at Hanford, WA. Images from ligo.caltech.edu.
In each of the LIGO observatories, a laser beam is split and travels both arms. The split laser beams bounce off mirrors placed at the end of each arm. The laser beams then return to the intersection of the arms. When gravitational waves reach Earth, they stretch the arms of the observatory, and this creates an incredibly small difference in the distances of the mirrors. LIGO detects that difference.
LIGO can detect a change in distance between its mirrors 1/10,000th the width of a proton.
LIGO can detect a change in distance between its mirrors 1/10,000th the width of a proton. This precision is equivalent to measuring the distance to the nearest star with a precision of the width of a human hair, or equivalent to measuring the expansion and contraction of our entire galaxy, the Milky Way, with the precision of the width of a thumb.
At least two detectors are needed in order to avoid false positives caused by local vibrations such as earthquakes, traffic, airplanes, or weather-related events, just to mention a few. The vast distance between the twin observatories is necessary to determine the direction of the source of gravitational waves, and also to verify that the signals come from space instead of from a local event.
Before LIGO’s detection system could be designed and constructed, physicists and astronomers needed to know what gravitational waves would look like and how they could be detected. Scientists relied on supercomputer simulations to predict the range of frequencies and intensities of gravitational waves. The input for the supercomputer simulations are the mathematical solutions of Einstein’s gravitational equations. The output of the simulations are the measurable characteristics of the waves, such as their intensity and frequency.
Some 40 years after Einstein published his gravitational equations for his theory of general relativity, a mathematical breakthrough came from Texas. Roy Kerr, a mathematics postdoctoral fellow at the University of Texas at Austin, found the mathematical solution for Einstein’s gravitational equation for a massive spinning object. In physics and astronomy, a spinning black hole is known as a Kerr black hole. Kerr’s mathematical work is the foundation of the current supercomputer simulations that led to the detection of gravitational waves by LIGO.
On September 14, 2015, LIGO’s detection system had barely finished being calibrated when a loud signal came through at the Livingston site. Data was streaming, and then: “Whoop.” Seven milliseconds later, a signal was detected at the Hanford site. After months of analyzing the data, which included noise elimination and detailed comparisons of signal predictions with computer simulations of massive objects in motion, the scientific team determined that the gravitational waves were generated by two black holes at a distance of 1.3 billion light years from us, somewhere beyond the galaxy of the Large Magellanic Cloud in the southern hemisphere sky.
The two black holes are 30 times as
massive as our sun.
The two black holes are 30 times as massive as our sun, circling each other at close to the speed of light before colliding and giving off an enormous amount of energy equivalent to about three solar masses. Comparing those three solar masses converted instead into visible light, they would be equivalent to the brightness of a billion trillion suns. In other words, the power received in the form of gravitational waves at LIGO is 50 times greater than the output of all the stars in the universe combined.
Just like sound and electromagnetic waves (light), gravitational waves cover a range of frequencies. Some of their frequencies overlap with the range of sound waves. In this sense we can hear gravitational waves. We can hear the universe. For centuries we have been observing the universe through light. Now we can listen to it.
Computational simulations had predicted that as two black holes spiral closer and closer in together, the frequency of the gravitational waves would generate increases. Scientists call these sounds “chirps,” because for humans those sounds would be like a bird’s chirp. It is truly amazing to hear the sounds recorded by LIGO. (See the video at the top of the article.)
There are massive objects other than black holes that are expected to generate gravitational waves. These include supernovas, pulsars, and neutron stars. The key observational parameter that tells us about the source of gravitational waves is the frequency of the waves. The frequency of the waves detected by LIGO indicated that the massive objects orbiting each other were black holes.
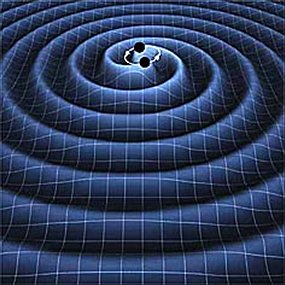
Gravitational waves produced by two orbiting black holes. K. Thorne (Caltech)/ T. Carnahan (NASA GSFC).
Dr. Reiner Weiss, Professor emeritus at MIT has offered this analogy:
Do you know what a glissando is? It’s when you run your fingers very quickly across the keys. If you started at the bottom of a keyboard and went all the way to the middle C and then hold that note for a little bit — that’s what this black hole signal happened to be….
There is a whole spectrum of gravitational waves. With LIGO, we’re looking for high-frequency waves. As to how high we can go, again, the piano analogy is a wonderful one. Because what we’re looking for are sources that go from the bottom of the piano to the top of the piano. They don’t stop at middle C, which is currently our detection limit with LIGO. They go higher.
Gravitational waves were indirectly
detected back in 1974.
Gravitational waves were not only predicted by Einstein in his theory of general relativity, but were also indirectly detected back in 1974 when Joseph Taylor, Jr., and Russell Hulse discovered a pulsar circling a neutron star and later observations showed that the pulsar’s orbit was shrinking in precise relation to the loss of energy due to gravitational waves described by Einstein’s general theory of relativity — a discovery that won Taylor and Hulse the 1993 Nobel Prize in Physics.
Some scientists consider the detection of gravitational waves to be as important as the 2012 discovery of the subatomic Higgs boson, sometimes called the “God particle.” Some consider it far more important, equating it to the moment when Galileo took up the telescope and looked at the planets in our solar system.
For centuries we have observed the universe only through light, which has limited us to a view of just 5% of the universe. Gravitational waves carry completely different information about phenomena in the universe. We have opened a new way of listening to a broadcasting channel which will allow us to discover phenomena we have never seen before.
We wave and we feel the wave: “Whooop.”
[Astronomer and artist Cecilia Colomé has a Ph.D. in Astrophysics from the University of Texas at Austin. She also edits mathematics and science textbooks and is a contributing editor to The Rag Blog where she coordinates The Rag Blog en español. Cecilia, also an accomplished visual artist, grew up in Mérida, the capital of the state of Yucatán, México, and now lives and works in Austin, Texas.]
Great article Cecilia!!!
Thank you
Like a binary black hole, your article is both awe-inspiring and formidable! 😉
Thanks for sharing Ceci.
Thank you dear Grace.
Good to see the RagBlog addressing a hard science I in which so much changes so fast (maybe not at the speed of light, but really fast 😄)
Your article is brilliant, Cecilia. Astrophysics made comprehensible even for English majors. Congratulations.
Thank you very much, Ray.
Very informative article on such a groundbreaking topic. Bravo Cecilia!